Anoxia and Euxinia Ocean Environmental Change
Reconstructing the history of euxinia in a coastal sea Caroline […]
Date Posted:
September 21, 2013
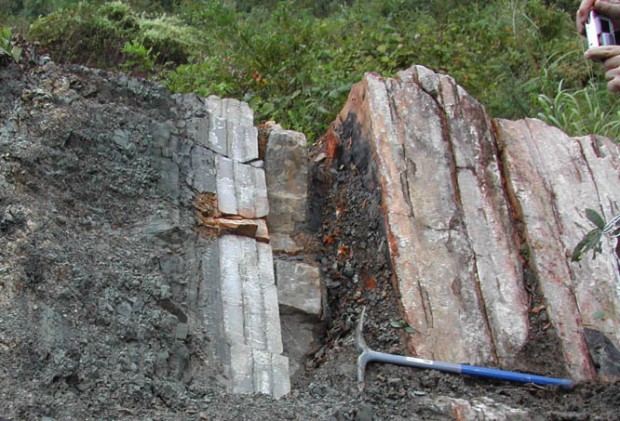
Reconstructing the history of euxinia in a coastal sea
Caroline P. Slomp, 2013 DOI 10.1130/focus0420131.1 Web http://geology.gsapubs.org/content/41/4/523.full
Areas of the coastal ocean where oxygen is low or absent in bottom waters, so-called dead zones, are expanding worldwide (Diaz and Rosenberg, 2008). Increased inputs of nutrients from land are enhancing algal blooms, and the sinking of this organic matter to the seafloor and subsequent decay leads to a high oxygen demand in bottom waters. Depending on the physical characteristics of the coastal system, this may initiate periodic or permanent water column anoxia and euxinia, with the latter term implying the presence of free sulfide (Kemp et al., 2009). Global warming is expected to exacerbate the situation, through its effects on oxygen solubility and water column stratification. In many modern coastal systems, anthropogenic changes are superimposed on natural variation and lack of knowledge of such variation makes the prediction of future changes in water column oxygen challenging (e.g., Grantham et al., 2004). That natural drivers alone can be the cause of widespread coastal anoxia is evident from studies of greenhouse periods in Earth’s past, including the oceanic anoxic events of the Cretaceous and Toarcian (Jenkyns, 2010).
Sediment proxy records are essential to any reconstruction of variations in anoxia and euxinia on time scales beyond several decades to a century. A variety of biological and geochemical indicators can be used for this purpose, such as the presence of the remains of benthic and pelagic organisms, laminations, biomarkers for eukaryotes or prokaryotes, and inorganic geochemical and mineralogical signatures in the sediment, and ideally, these methods are combined. Sediments that are deposited below a euxinic water column are, for example, typically enriched in organic carbon, sulfur, iron, and trace metals such as rhenium and molybdenum (Gooday et al., 2009). Recent additions to this paleo-redox toolbox are the isotope systems of Fe and Mo (Lyons et al., 2009). Reconstruction of the temporal changes in the oxic-anoxic interface (chemocline) in the water column forms a key step in the identification of the external drivers and internal feedbacks that contribute to anoxia and euxinia in a given system. In their study of sediments from the Black Sea, Eckert et al. (2013, p. 431 in this issue of Geology), make this step by providing, for the first time, a basin-wide reconstruction of the evolution of the chemocline in this silled coastal basin over the Holocene.
Silled basins in humid areas such as Kau Bay (Indonesia), the Baltic Sea, and the Black Sea, are particularly sensitive to low oxygen conditions because of salinity stratification and associated reduced vertical mixing (Kemp et al., 2009). All these inland seas have an intriguing history and were originally coastal lakes that were transformed to marine basins due to postglacial sea-level rise. Kau Bay is only semi-euxinic, and is subject to incursions of low-oxygen non-sulfidic bottom waters that alternate with periods of anoxic, sulfidic bottom waters (Middelburg et al., 1991). The Baltic Sea also alternates between redox states: it experienced various periods of low oxygen over the Holocene, but is currently subject to a human-induced period of anoxia, with its bottom waters largely oxic around 1900 CE (Conley et al., 2009). The Black Sea is the largest euxinic basin in the world and differs in being permanently euxinic. This is the result of the strong stratification that developed after its fore-runner fresh water lake became connected to the Mediterranean Sea through the narrow, shallow Straits of the Bosporus at ca. 9 kyr B.P. Water column anoxia developed across the deep basin from ca. 7.5 kyr B.P. onward (Degens and Ross, 1974), and the chemocline is presently located at ∼100 m depth.
Strong variations in the geochemical and paleo-ecological composition and genetic signature of the sediments in the Black Sea provide testimony that the conditions in the water column have been far from constant over the past ∼7.5 kyr. Two phases of deposition are generally distinguished based on visual characteristics of the sediments. Following the onset of anoxia, a finely laminated, dark, organic-rich sediment layer formed first (Unit II), followed by deposition of alternating microlaminae of calcareous (white) and organic- and clay-rich material (black) from ca. 2.6 kyr B.P. to the present (Unit I). The shift from Unit II to Unit I was originally attributed to the invasion of the coccolithophore Emiliania huxleyi when salinity rose above 11 (Arthur and Dean, 1998). However, genetic analyses show that this calcifying haptophyte colonized the photic zone of the Black Sea shortly after the connection to the Bosporus, and the Unit I–II transition marks the moment that coccoliths began to be preserved in the sediments (Coolen et al., 2009).
The delayed appearance of Unit II on the slopes of the basin has been taken as an indicator of a slow rise of the chemocline following the onset of anoxia (Degens and Ross, 1974). The rise was fast enough, however, for the chemocline to reach the photic zone by the time of deposition of the lower part of Unit II, as indicated by the presence of biomarkers for photosynthetic green sulfur bacteria (Sinnighe Damsté et al., 1993; Repeta, 1993). Results of similar analyses for the upper part of Unit II suggested a subsequent descent of the chemocline followed by re-establishment in the photic zone during deposition of Unit I. At the time, controversy remained about the temporal and spatial variability in the position of the chemocline, and the extent to which the water column and photic zone remained euxinic throughout deposition of Units I and II. This debate was partially resolved when Wilkin et al. (1997) showed that the size of the pyrite framboids in Units I and II were in line with a continuously euxinic water column. Using a composite record of sediment Fe, Mo, and Fe-isotopes derived from data for nine sites throughout the basin, Eckert et al. (2013) now confirm the evolution of Black Sea euxinia, as suggested in these earlier studies, and provide a more consistent and basin-wide timing for the series of events.
The variation in strength of the ‘Fe shuttle’ forms the heart of their reconstruction. This term is used to describe the lateral transfer of Fe released from suboxic shelf sediments to the deep basin. The authors use their Fe/Al record as a direct indicator of the position of the chemocline, where low Fe/Al indicates a weak shuttle with a chemocline impinging on the slope. A high Fe/Al, in contrast, indicates a chemocline allowing suboxic water to spread over part of the shelf and supporting an intense Fe shuttle. The authors also make use of the fact that Mo data can be used to reconstruct the hydrography of a basin, which for the Black Sea allows an estimate of the inflow of Mediterranean seawater. Fe isotope analyses bolster the argument for the shelf-source of Fe. The emerging timeline is as follows (Unit II): (1) a gradual rise of the chemocline over a period of ∼2 kyr following the onset of anoxia at ca. 7.6 kyr B.P., (2) fully developed euxinic conditions with an ascent of the chemocline onto the shelf at ca. 5.3 kyr B.P., (3) a subsequent descent of the chemocline, and (Unit I) (4) establishment of the chemocline in its present-day position at the shelf break from 2.7 kyr B.P. onward.
But this is not the full story. Besides a good timeline for euxinia in the Black Sea, we need to understand the hydrographic and biogeochemical processes that drove these changes in redox conditions, and there much work still needs to be done. The evolution of the salinity in the basin, for example, is not well constrained. Recent qualitative reconstructions of salinity based on various proxies suggest that values of surface water salinity in the Black Sea rose until ca. 3 kyr B.P., followed by a gradual freshening to present-day values (van der Meer et al., 2008; Coolen, 2011). Possible causes for the freshening include an increase in fluvial discharge and decreased evaporation (Giosan et al., 2012). An associated increase in stratification may have contributed to the shallowing of the chemocline at the onset of the deposition of Unit I. Also, the processes leading to the increased total organic carbon (TOC) in Unit II as compared to the overlying and underlying units are not well understood. The high TOC is frequently interpreted as an indicator of enhanced nutrient availability and productivity following the inflow of Mediterranean seawater, and transition of limnic (oxic) to marine (anoxic and euxinic) conditions (also see Eckert et al., 2013). However, the sources of the nutrients fuelling this productivity have not been identified and whether, for example, phosphorus release from sediments or river water is more important is still an open question. Finally, the cause of the descent of the chemocline after ca. 5.3 kyr B.P. remains unknown. While Eckert et al. (2013)propose a decreased seawater input or increased river input as potential causes,van der Meer et al. (2008), in contrast, suggest that the absence of a shallow chemocline can be best explained by the high sea-surface salinity at the time.
Despite the open questions, the Eckert et al. (2013) study is important because it provides a more solid timeline and integrated view of the evolution of euxinia in the Black Sea, which is highly useful for assessments of climatic and other drivers of temporal change. The tools used can also be applied to better interpret sediment records from other marine systems, both modern and ancient, and can thereby aid in the assessment of the time scales of a possible decline into, and recovery from, wide-scale anoxia and euxinia. Such knowledge is important in a warming world where water column deoxygenation in the coastal zone is becoming more and more common.
Ocean acidification and global oceanic euxinia
SILVA-TAMAYO, Juan Carlos, PAYNE, Jonathan L., WIGNALL, Paul, NEWTON, Robert J., WEYER, Stefan, EISENHAUER, Anton, MAHER, Kate, NEUBERT, Nadja, and LAU, Kimberly V., 2013 https://gsa.confex.com/gsa/2013AM/webprogram/Paper229367.html
The most catastrophic extinction event in the history of animal life occurred at the end of the Permian Period, ca. 252 Mya. Ocean acidification and global oceanic euxinia have each been proposed as causes of this biotic crisis, but the magnitude and timing of change in global ocean chemistry remains poorly constrained.
Here we use Ca, Mo and U isotopes applied to globally distributed, well dated late Permian- early Triassic sedimentary sections to better constrain the magnitude and timing of change in ocean chemistry through this interval. All the investigated carbonate successions (Turkey, Italy and China) exhibit decreasing d44/40Ca compositions, paralleling a major decrease in d13C values. These findings support an episode of ocean acidification coincident with the major biotic crisis. The Mo and U isotope records exhibit significant rapid negative anomalies at the onset of the main extinction interval, suggesting rapid expansion of anoxic and euxinic marine bottom waters during the extinction interval. The rapidity of the isotope excursions in Mo and U suggests substantially reduced residence times of these elements in seawater relative to the modern, consistent with expectations for a time of widespread anoxia. The large C-isotope variability during the early Triassic, which is similar to that of the early-middle Cambrian, suggests imply largely biogenetically controlled perturbations of the oceanic carbon cycle. These findings strengthen the evidence for a global ocean acidification event coupled with rapid expansion of anoxic zones as drivers of end-Permian extinction in the oceans.
Massive release of hydrogen sulfide to the surface ocean and atmosphere during intervals of oceanic anoxia
Lee R. Kump, Alexander Pavlov and Michael A. Arthur DOI: 10.1130/G21295.1 http://geology.gsapubs.org/content/33/5/397.full
Simple calculations show that if deep-water H2S concentrations increased beyond a critical threshold during oceanic anoxic intervals of Earth history, the chemocline separating sulfidic deep waters from oxygenated surface waters could have risen abruptly to the ocean surface (a chemocline upward excursion). Atmospheric photochemical modeling indicates that resulting fluxes of H2S to the atmosphere (>2000 times the small modern flux from volcanoes) would likely have led to toxic levels of H2S in the atmosphere. Moreover, the ozone shield would have been destroyed, and methane levels would have risen to >100 ppm. We thus propose (1) chemocline upward excursion as a kill mechanism during the end-Permian, Late Devonian, and Cenomanian–Turonian extinctions, and (2) persistently high atmospheric H2S levels as a factor that impeded evolution of eukaryotic life on land during the Proterozoic.
Conditions required for oceanic anoxia/euxinia: Constraints from a one-dimensional ocean biogeochemical cycle model
Kazumi Ozaki, Shigeo Tajima, Eiichi Tajika http://www-sys.eps.s.u-tokyo.ac.jp/~tajika/papers/Ozaki%20et%20al%20(2011)%20EPSL.pdf
Widespread black shale depositional intervals termed oceanic anoxic events (OAEs) occurred repeatedly during the Phanerozoic Eon. Here we developed a new vertical one-dimensional ocean biogeochemical cycle model that involves several chemical reactions in an oxic–anoxic–sulfidic water column. To explore the theoretical constraints for global oceanic anoxia/euxinia quantitatively and systematically, we conducted sensitivity analyses of the proposed causal mechanisms, including elevated rates of riverine phosphorus (P) input, ocean stagnation, and lowered oxygen solubility due to climate warming. We gave special attention to the vertical chemical structure of the ocean and also to the characteristic behaviors of the marine P cycle under anoxic conditions, because the relationship between the depth of anoxia and the benthic phosphorus flux could be important for the occurrence of oceanic anoxia/euxinia. Steady-state simulations indicated that (1) a decrease in ocean stagnation or oxygen solubility is not enough by itself to achieve widespread anoxia with the present reactive P river input rate, and (2) shallow water anoxia followed by massive P liberation from surface sediments can lead to widespread eutrophication and anoxia/euxinia. We conclude that elevated riverine flux of reactive P is the most important factor for triggering global anoxic events via a positive feedback loop among ocean anoxia, phosphorus regeneration, and surface biological productivity.
Photic zone euxinia during the permian-triassic superanoxic event
Kliti Grice, Changqun Cao, Gordon D Love, Michael E Böttcher, Richard J Twitchett, Emmanuelle Grosjean, Roger E Summons, Steven C Turgeon, 2005 DOI:10.1126/science.1104323 http://www.researchgate.net/publication/8069528_Photic_zone_euxinia_during_the_Permian-triassic_superanoxic_event
Carbon and sulfur isotopic data, together with biomarker and iron speciation analyses of the Hovea-3 core that was drilled in the Perth Basin, Western Australia, indicate that euxinic conditions prevailed in the paleowater column during the Permian-Triassic superanoxic event. Biomarkers diagnostic for anoxygenic photosynthesis by Chlorobiaceae are particularly abundant at the boundary and into the Early Triassic. Similar conditions prevailed in the contemporaneous seas off South China. Our evidence for widespread photiczone euxinic conditions suggests that sulfide toxicity was a driver of the extinction and a factor in the protracted recovery.
δ 13C evidence that high primary productivity delayed recovery from end-Permian mass extinction
K.M. Meyer a, ⁎, M. Yub , A.B. Jost a , B.M. Kelley a , J.L. Payne http://siberia.mit.edu/sites/siberia.mit.edu/files/articles_news_press/Meyer-Payne-EPSL-2011.pdf
K.M. Meyer a, ⁎, M. Yub , A.B. Jost a , B.M. Kelley a , J.L. PEuxinia was widespread during and after the end-Permian mass extinction and is commonly cited as an explanation for delayed biotic recovery during Early Triassic time. This anoxic, sulfidic episode has been ascribed to both low- and high-productivity states in the marine water column, leaving the causes of euxinia and the mechanisms underlying delayed recovery poorly understood. Here we use isotopic analysis to examine the changing chemical structure of the water column through the recovery interval and thereby better constrain paleoproductivity. The δ 13C of limestones from 5 stratigraphic sections in south China displays a negative gradient of approximately 4‰ from shallow-to-deep water facies within the Lower Triassic. This intense gradient declines within Spathian and lowermost Middle Triassic strata, coincident with accelerated biotic recovery and carbon cycle stabilization. Model simulations show that high nutrient levels and a vigorous biological pump are required to sustain such a large gradient in δ 13C, indicating that Early Triassic ocean anoxia and delayed recovery of benthic animal ecosystems resulted from too much productivity rather than too little.
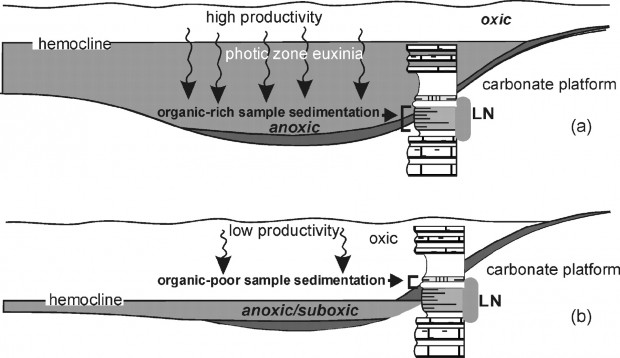
Major perturbation of ocean chemistry and a ‘Strangelove Ocean’ after the end-Permian mass extinction
Michael R. Rampino and Ken Caldeira, 2005 DOI: 3121.2005.00648.x http://pubs.giss.nasa.gov/docs/2005/2005_Rampino_Caldeira_1.pdf
The severe mass extinction of marine and terrestrial organisms at the end of the Permian Period (c. 251 Ma) was accompanied by a rapid (<100 000 years and possibly <10 000 years) negative excursion of c. 3& in the d 13C of the global surface oceans and atmosphere that persisted for some 500 000 years into the Early Triassic. Simulations with an ocean–atmosphere/ carbon-cycle model suggest that the isotope excursion can be explained by collapse of ocean primary productivity, and changes in the delivery and cycling of carbon in the oceans and on land. Model results suggest that severe reduction of marine productivity led to an increase in surface-ocean dissolved inorganic carbon and a rapid, short-term increase in atmospheric pCO2 (from a Late Permian base of 850 ppm to c. 2500 ppm). Increase in surface ocean alkalinity may have stimulated the widespread microbial and abiotic shallow-water carbonate deposition seen in the earliest Triassic. The model is also consistent with a long-term (>1 Ma) decrease in sedimentary burial of organic carbon in the early Triassic.’ after the end-Permian mass extinction.
Oceanic Euxinia in Earth History: Causes and Consequences
Katja M. Meyer and Lee R. Kump, 2008 DOI: 10.1146/annurev.earth.36.031207.124256 http://www.annualreviews.org/doi/abs/10.1146/annurev.earth.36.031207.124256
Euxinic ocean conditions accompanied significant events in Earth history, including several Phanerozoic biotic crises. By critically examining modern and ancient euxinic environments and the range of hypotheses for these sulfidic episodes, we elucidate the primary factors that influenced the generation of euxinia. We conclude that periods of global warmth promoted anoxia because of reduced solubility of oxygen, not because of ocean stagnation. Anoxia led to phosphate release from sediments, and continental configurations with expansive nutrient-trapping regions focused nutrient recycling and increased regional nutrient buildup. This great nutrient supply would have fueled high biological productivity and oxygen demand, enhancing oxygen depletion and sulfide buildup via sulfate reduction. As long as warm conditions prevailed, these positive feedbacks sustained euxinic conditions. In rare, extreme cases, euxinia led to biotic crises, a hypothesis best supported by evidence from the end-Permian mass extinction.
Relevant
A new model for Proterozoic ocean chemistry
D. E. Canfield, 1998 doi:10.1038/24839 http://ebme.marine.rutgers.edu/HistoryEarthSystems/HistEarthSystems_Fall2008/Week5b/Canfield_Nature_1998.pdf
There was a significant oxidation of the Earth’s surface around 2 billion years ago (2 Gyr)1, 2, 3, 4. Direct evidence for this oxidation comes, mostly, from geological records of the redox-sensitive elements Fe and U reflecting the conditions prevailing during weathering1, 2, 3. The oxidation event was probably driven by an increased input of oxygen to the atmosphere arising from an increased sedimentary burial of organic matter between 2.3 and 2.0 Gyr5. This episode was postdated by the final large precipitation of banded iron formations around 1.8 Gyr1, 2. It is generally believed that banded iron formations precipitated from an ocean whose bottom waters contained significant concentrations of dissolved ferrous iron, and that this sedimentation process terminated when aerobic bottom waters developed, oxidizing the iron and thus removing it from solution1, 2. In contrast, I argue here that anoxic bottom waters probably persisted until well after the deposition of banded iron formations ceased; I also propose that sulphide, rather than oxygen, was responsible for removing iron from deep ocean water. The sulphur-isotope record supports this hypothesis as it indicates increasing concentrations of oceanic sulphate, starting around 2.3 Gyr6, leading to increasing rates of sulphide production by sulphate reduction. The increase in sulphide production became sufficient, around 1.8 Gyr, to precipitate the total flux of iron into the oceans. I suggest that aerobic deep-ocean waters did not develop until the Neoproterozoic era (1.0 to 0.54 Gyr), in association with a second large oxidation of the Earth’s surface. This new model is consistent with the emerging view of Precambrian sulphur geochemistry and the chemical events leading to the evolution of animals, and it is fully testable by detailed geochemical analyses of preserved deep-water marine sediments.
Interpreting carbon-isotope excursions: Stranglove oceans
Lee R. Kump, 1991 DOI:10.1130/0091-7613(1991)0192.3.CO;2 http://www.researchgate.net/publication/249519735_Interpreting_carbon-isotope_excursions_Stranglove_oceans
Large negative excursions in marine carbonate δ13C are commonly associated with period boundaries and mass extinctions. Explanations for these events must be consistent with limitations imposed by carbon-isotope mass balance. At steady state (i.e., for excursions lasting more than 105 yr), the surface ocean δ13C is set by the organic fraction of the total carbon burial rate and the magnitude of the photosynthetic isotope effect. The δ13C of the deep ocean and the surface-to-deep isotope gradient are set by both the organic fraction of the ocean’s remineralized particulate flux and the magnitude of the isotope effect. Thus it is the carbon-isotope composition of the deep ocean that is most reflective of internal oceanic processes; the surface ocean records changes in the longer term throughput of carbon in the system. The cessation of organic export from the surface ocean, such as is presumed to have caused the Strangelove ocean condition of the Cretaceous/Tertiary (K/T) boundary, leads to an isotopically homogeneous ocean in decades to centuries. If this condition persists, the ocean’s isotopic composition approaches that of the riverine weathering input (in 105 yr). Failure to approach this value during the K/T event suggests continued production and burial of organic carbon, dominantly in either terrestrial or shallow-marine environments.
Rapid expansion of oceanic anoxia immediately before the end-Permian mass extinction
Gregory A. Brennecka, Achim D. Herrmanna, Thomas J. Algeo, and Ariel D. Anbara, 2011 http://www.pnas.org/content/early/2011/10/05/1106039108.full.pdf
Periods of oceanic anoxia have had a major influence on the evolutionary history of Earth and are often contemporaneous with mass extinction events. Changes in global (as opposed to local) redox conditions can be potentially evaluated using U system proxies. The intensity and timing of oceanic redox changes associated with the end-Permian extinction horizon (EH) were assessed from variations in 238U∕ 235U (δ 238U) and Th/U ratios in a carbonate section at Dawen in southern China. The EH is characterized by shifts toward lower δ 238U values (from −0.37‰ to −0.65‰), indicative of an expansion of oceanic anoxia, and higher Th/U ratios (from 0.06 to 0.42), indicative of drawdown of U concentrations in seawater. Using a mass balance model, we estimate that this isotopic shift represents a sixfold increase in the flux of U to anoxic facies, implying a corresponding increase in the extent of oceanic anoxia. The intensification of oceanic anoxia coincided with, or slightly preceded, the EH and persisted for an interval of at least 40,000 to 50,000 y following the EH. These findings challenge previous hypotheses of an extended period of whole-ocean anoxia prior to the end-Permian extinction.
Biogeochemistry of oceanic euxinia in Earth history: Numerical modeling and evaluation of biomarkers using modern analogs
K M Meyer, 2008 DOI: 10.1146/annurev.earth.36.031207.124256 http://udini.proquest.com/view/biogeochemistry-of-oceanic-euxinia-goid:849724677/
The present study is a multi-disciplinary exploration of the overarching factors that contribute to oceanic euxinia and its sedimentary signature. Using the GENIE Earth system model, I investigated the physical and chemical factors that enhance euxinia in the geological record. Sustained global warmth, ocean circulation patterns replete with nutrient-trapping geographies, and elevated nutrient conditions initiate biological feedbacks that stimulate euxinia based on this numerical analysis. I then use the example of the end-Permian, an interval during which euxinia and mass extinction coincide, to further explore the biogeochemical controls on the buildup of sulfide in the global ocean. With the end-Permian configuration of GENIE, I examine the distribution of H 2 S accumulation in the ocean and quantify the impact of anoxygenic phototrophy in reducing surface-water [H2 S] under a range of oceanic phosphate conditions. The modeled spatial predictions largely agree with the geological record of geochemical and biotic change across the Permian-Triassic boundary. Addition of a nitrogen cycle to GENIE results in further reduction of hydrogen sulfide buildup. The high productivity euxinic ocean is N-limited as evidenced by low N:P ratios in the deep ocean.
This work serves as a quantitative basis for linking the fossil record of extinction to the geochemical record of carbon and sulfur cycle perturbation. The geologically stable remnants of carotenoids from the anoxygenic green and purple sulfur bacteria are a powerful method of identifying photic zone euxinia in the rock record. I used pigment and clone library analyses from planktonic and benthic samples collected in a microbially dominated meromictic lake (Fayetteville Green Lake, New York) to test the hypothesis that the purple sulfur bacterial carotenoid, okenone, is only produced in planktonic settings. We report evidence of okenone and purple sulfur bacteria in both benthic and planktonic environments of Fayetteville Green Lake. This finding highlights the need for continued study of modern environments and the physiology of carotenoid expression in modern microbes to aid interpretation of biomarkers for photic zone euxinia in the geological record.
Further Reading
Earth: The Operators’ Manual (Part on hydrogen sulfide), Richard B. Alley
Wiktionary
Euxinic (comparative more euxinic, superlative most euxinic)
- Having restricted hydrologic circulation, leading to stagnant or anaerobic conditions
Related terms
Euxinia
Related
Ocean Acidification in Earth’s Past: Insights to the Future – James Zachos
[…] Anoxic and Euxinia Ocean Environmental Change http://climatestate.com/2013/09/21/eu… […]