Methane release from the East Siberian Arctic Shelf and the Potential for Abrupt Climate Change
Russian Academy of Sciences, Far Eastern Branch, Pacific Oceanological Institute, Vladivostok, Russia "Opening the Arctic", Washington, Nov.30th-Dec.2nd, 2010.
Natalia Shakhova, Igor Semiletov | Source | University of Alaska, Fairbanks, International Arctic Research Centre, USA
Outline
- What do we know about methane potential of the ESAS?
- Is there a mechanism responsible for transformation of methane potential to modern methane source?
- What are triggers forcing non-gradual modeof methane emission from the seabed?
- Is there a plausible mechanism, which could determine massive and abrupt methane releases?
- Why is the ESAS unique in its ability to accommodate huge methane potential?
The East Siberian Arctic ESAS Shelf (ESAS)
The ESAS is the most extensive and the shallowest shelf of the World Ocean
- 2.1×106 km2 area (~25% of the Arctic Shelf, ~8% of the total area of the World Ocean’s continental shelf)
- ~75% is shallower than 50 m (mean depth of the continental shelf is 130 m);this provides very short conduit for methane to escape to the atmosphere with almost no oxidation.
The ESAS provides favorable conditions for methanogenesis and accumulation of natural hydrocarbons
The highest rates of carbon rain to the bottom provides required amount of organic carbon to be buried in the seabed; Annual sediment accumulation is about 10×10 ^12 g C yr-^1, which approximately equals the amount of sediment accumulated over the entire pelagic zone of the World Ocean.
Stable conditions of the continental margin lead to formation of very thick sedimentary basins (up to 20 km thick), which provide temperature and pressure conditions required for methane production
Geological conditions are favorable for formation of all types of seabed deposits: extensive coal beds, natural gas and oil fields; hydrate accumulations within and out of gas hydrate stability zone (GHSZ).
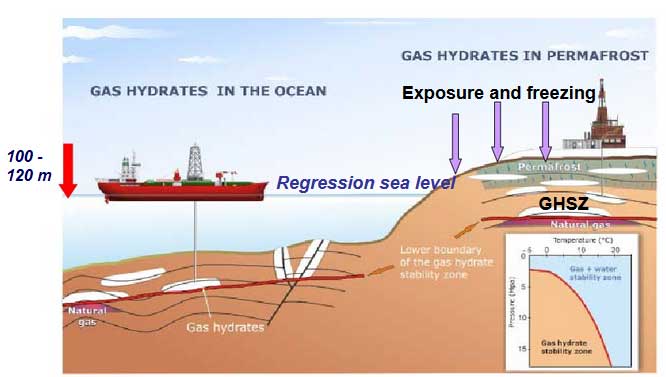
Upward moving geofluids provide transport of hydrocarbons to the areas of gas hydrate stability zone (GHSZ).
Arctic shelf hydrate formation: Cold climate epoch
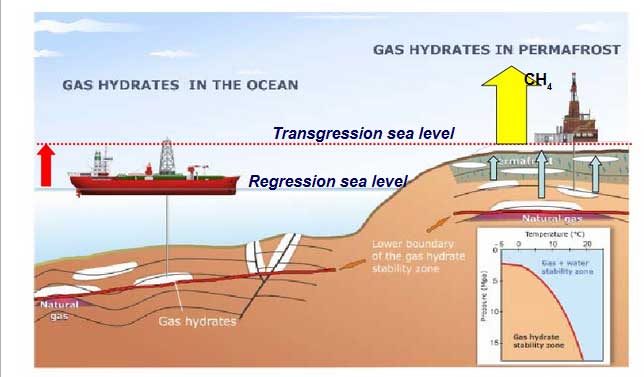
Arctic shelf hydrate destabilization: Warm climate epoch
Stability of sub-sea permafrost is key to stability of permafrost-related hydrate deposits
Specific features of the Arctic shelf methane hydrates
- More sensitive to warming, because only 1/3 of energy required to convert deep ocean hydrates to free gas (54.2 kJ/mol) requires to convert to free gas Arctic hydrates (18,2kJ/mol)
- More vulnerable because they have naturally been experiencing warming by as much as 17˚C while deep oceanic hydrates were warmed by less than 1˚C
- More significant in their accumulations, because their spatial concentration is many folds greater as well as pore occupancy (20-100% vs. 1-2% of deep oceanic hydrates)
- More potential for abrupt releases: Long-lasting permafrost impermeability determines huge potential of postponed emissions (like a pressure-cooker keeps all the steam inside till you release).
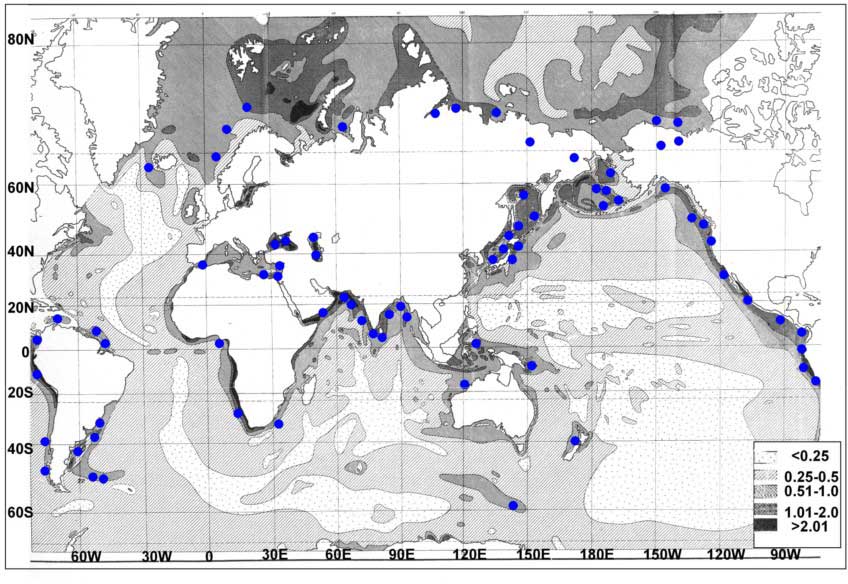
Significance of Arctic shelf hydrates vs. hydrate accumulations in the deep ocean
Hydrates are found here C org in sediments is highest.
What is known about mechanisms of permafrost destabilization associated with formation of gas migration pathways?
There are two basic mechanisms of permafrost destabilization:
1) Natural process of permafrost equilibration with its warmer environment determines talik formation (gradual process) the process is ongoing faster in the fault zones.
2) Natural process of tectonic and seismic activity in the region (non-gradual process) causes permafrost breaks; the process is occurring wherever such events take place.
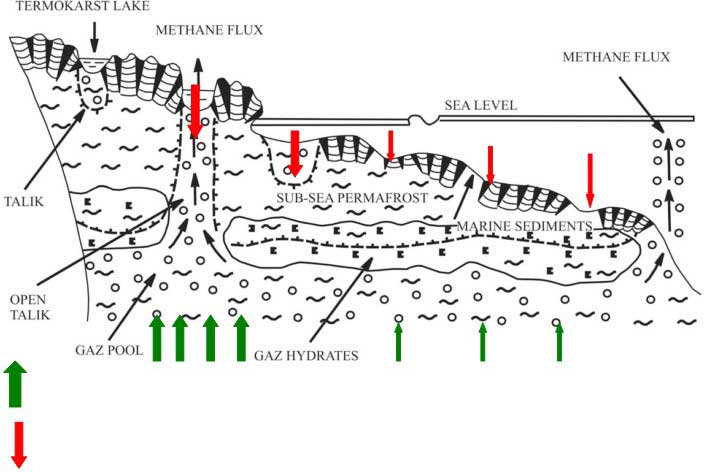
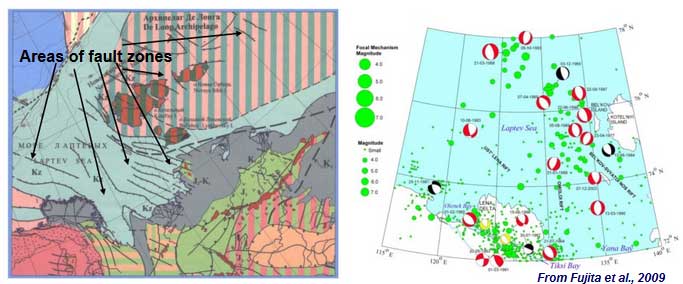
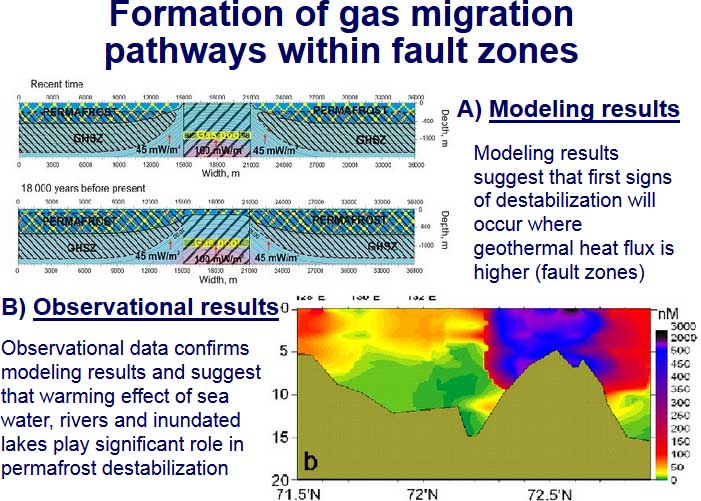
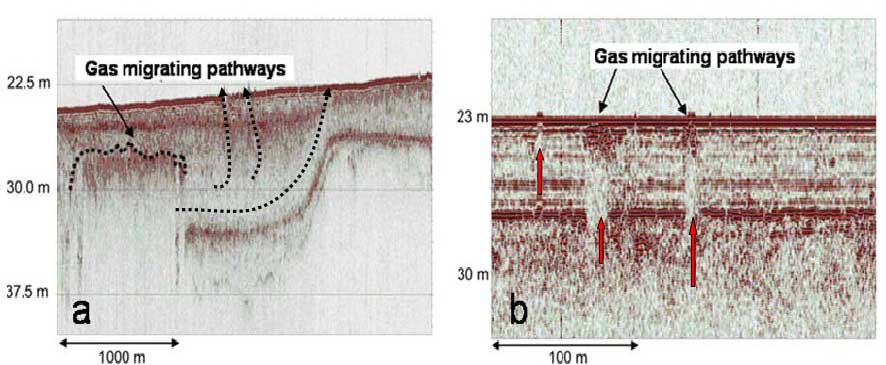
- Mechanism of combined talik formation(geothermal heat + thermokarst)
- Most of the ESAS area is affected by tectonic and seismic activities
- Formation of gas migration pathways within fault zones
- Over pressured gas fronts serve as a powerful geological force to build up migration pathways
- Pingo-like features as a gas migration pathway
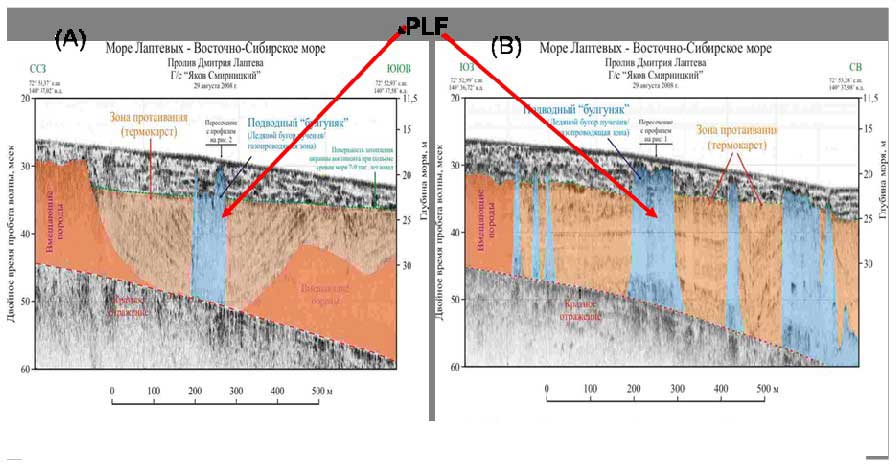
How far has permafrost destabilization gone by now?
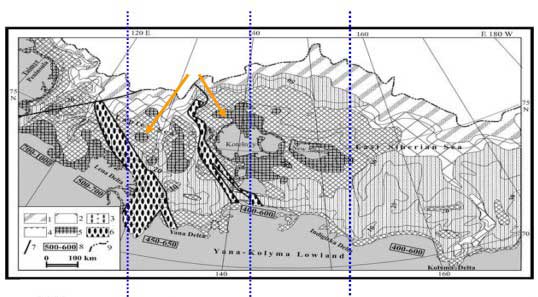

Manifestations of methane release from the ESAS
Methane release in the winter
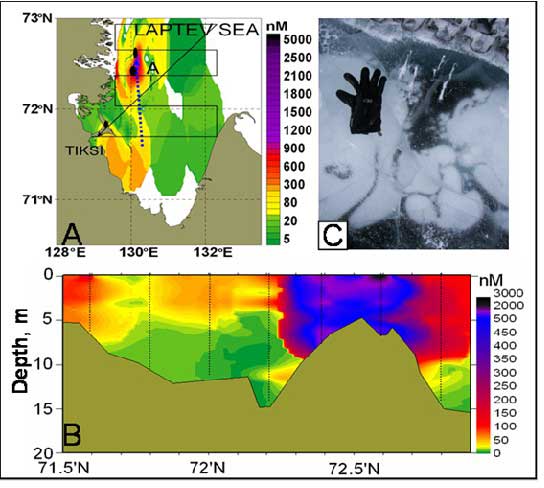
Non-gradual emission mode determines strong methane pulses to the atmosphere
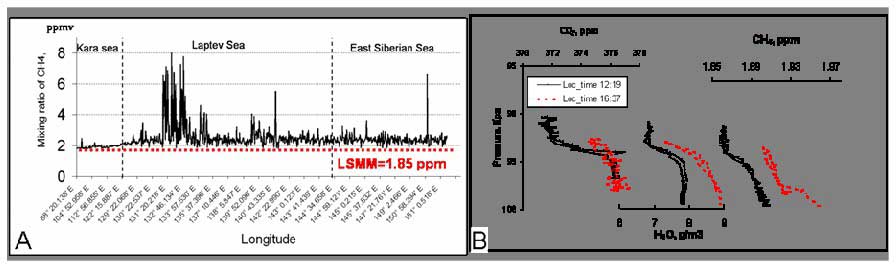
Acceleration mechanisms
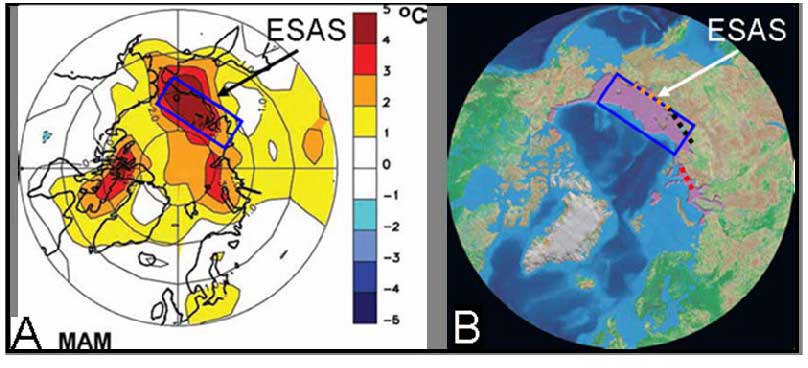
A) Observed warming on the ESAS (March-April-May; MAM, 2000-2005 versus 1970-1999, NOAA) is the strongest in the entire Arctic and the region is now 5˚C warmer compared with average springtime temperature registered during the 20th century;
B) Circum-Arctic map of sub-sea permafrost (shown in purple) (ACIA, 2005). This compilation suggests that most (~ 80%) of the relict submarine permafrost is predicted on the ESAS;
Warming effect of Arctic rivers runoff is increasing

Area of open water in winter is increasing drastically
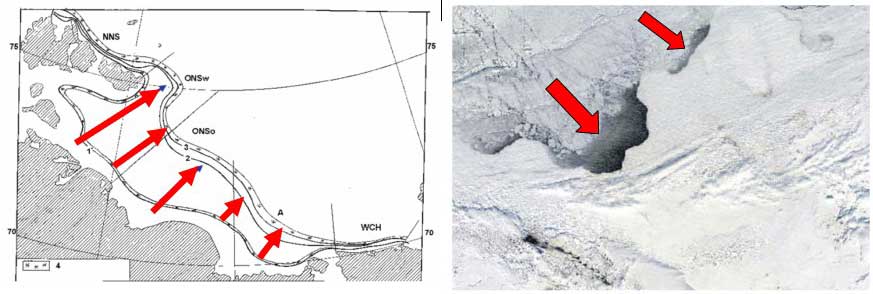
During the last two decades areas of flaw polynyas in the ESAS increased 5 times
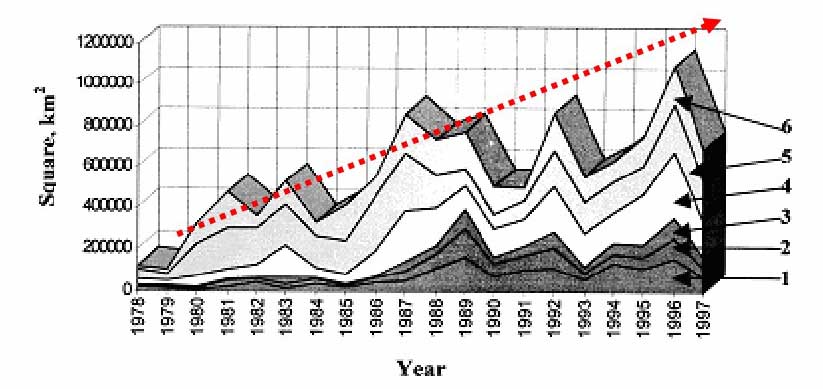
Additional factors serving to enhance permafrost destabilization in the ESAS
- Decreasing sea ice extent
- Change in hydrological pattern
- increasing frequency of high speed winds
- increasing frequency of deep convection events (mixing to the bottom) during summer
- Warming of bottom water (up to 3˚C during the last three decades)
Recent modeling results suggest wide scale sub-sea permafrost disintegration
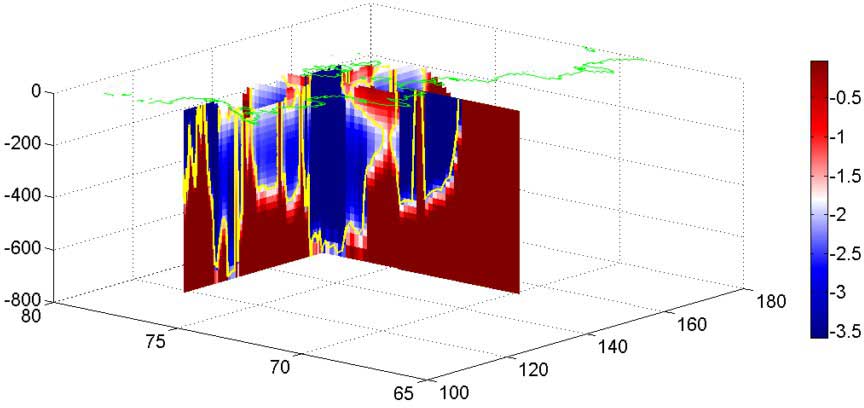
How much is the methane potential of the ESAS?
Accumulated methane potential of the ESAS:
- 1) Corg in permafrost ~500 Gt
- 2) Methane accumulation in hydrate deposits(GHSZ=100m) ~1000 Gt
- 3) Free gas beneath the GHSZ ~700 Gt OR Postponed emissions:1 year – 8Tg (Tg=1012 g); 1000 years – 8Gt(Gt=1015g); 90 000 years – 720 Gt
Conservative estimates
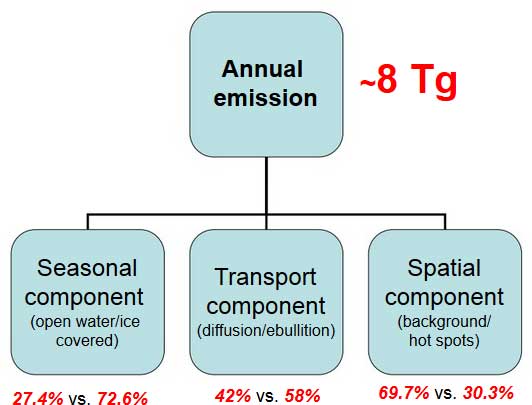
Directly observed fluxes exceed estimated by up 3 orders of magnitude
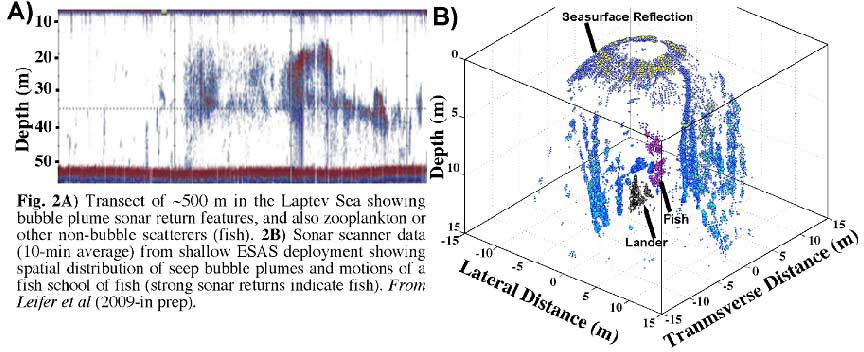
Example from the Trofimov seep field
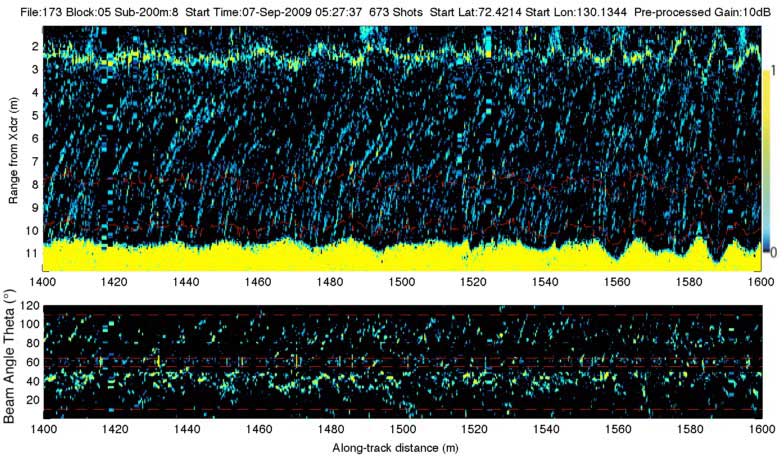
Map of the Trofimov seep field offshore of the Trofimov Channels,Lena River Delta
Conclusions
Permafrost failure uncorks huge gas reservoir, leading to large scale releases of methane from seabed.
Methane emissions from the ESAS depend on occurrence of gas migration pathways, developing through permafrost body (open taliks; permafrost breaks, discontinuity in sediment layers, fault zones, sediment settlement and adjustment etc.); this determines non-gradual emission mode. Given that the ESAS is tectonically and seismically active area, seismic events (including endogenous seismicity) might cause abrupt methane releases.
Studying the current state of sub-sea permafrost is of critical importance in order to elucidate the time scale of the ongoing process. Given that spatial and temporal variability of methane releases is very high, this underscores importance of establishing monitoring network over the ESAS. Considering the significance of the ESAS methane reservoir and enhancing mechanism of its destabilization, this region should be considered the most potential in terms of possible climate change caused by abrupt release of methane.
Further reading
Fault zones as a primary pathways for methane leakage from seabed deposits over the East Siberian Arctic Shelf
Shakhova, Natalia; Romanovskii, Nicolai | Source Harvard / NASA (2010)
Abstract
Recent data suggest the subsea permafrost currently is experiencing significant changes in its thermal regime. For instance, the temperature of subsea permafrost measured in deep boreholes (down to 70 m) 20-km offshore the NW Lena delta was as warm as -1°C, while in the same area terrestrial permafrost had a much lower annual mean temperature (about -12°C) (Rachold et al 2006). At such elevated temperatures, saline permafrost sediments are unfrozen, providing multiple potential CH4 migration pathways. Available data suggest the ESAS subsea permafrost is leaking substantial CH4. Geophysical data identified numerous gas seeps, mostly in depressions above prominent reflectors, underscoring the likelihood that subsea permafrost is a CH4 source to the overlying water and atmosphere. Numerical models predict permafrost destabilization 5-10 kYr after inundation, depending on the duration of inundation relative to the duration of previous freezing (Soloviev et al 1987), while current predicted open talik (i.e., thawed sediment within permafrost that permit CH¬4 migration) distributions comprise only up to 10% of the ESAS area (Romanovskii et al 2005). Recent observational data go in good agreement with these results as they suggest that ~10% of the ESAS seabed serves as a strong CH¬4 source, composing plume areas and allowing majority of aqueous CH4 (both dissolved and bubbles) to propagate through the water column and escape to the atmosphere. Emissions from plume areas contribute up to 60% of the total annual methane flux from the ESAS.
On carbon transport and fate in the East Siberian Arctic land–shelf–atmosphere system
Igor P Semiletov, Natalia E Shakhova, Valentin I Sergienko, Irina I Pipko and Oleg V Dudarev | Source IOP Science (2012)
Abstract
This review paper summarizes current understanding of the transport of organic carbon to, and the fate of organic carbon within, the East Siberian Arctic Shelf (ESAS), and of processes determining carbon dioxide (CO2) and methane (CH4) fluxes from the ESAS to the atmosphere achieved from analyzing the data sets obtained on 20 expeditions performed from 1999 to 2011. This study of the ESAS was aimed at investigating how redistribution of old carbon from degrading terrestrial and sub-sea permafrost and from coastal erosion contributes to the carbon pool of the ESAS, how changes in the hydrological cycle of the surrounding land and alteration of terrestrial carbon cycles affect the hydrological and biogeochemical parameters of shelf water masses, and which factors control CH4 and CO2 emissions from the ESAS. This report describes selected results achieved by a developing international scientific partnership that has been crucial at every stage of the study and will be even more important in the future.
Response of methane sources to rapid Arctic warming (July 2013)
About the Author: Climate State
